Carbon Unleashed: Harnessing Thermal Energy Storage
Hot energy storage to keep our climate cool
As we bask in the warmth of Labor Day weekend, let's explore the world of thermal energy storage. Let’s discover graphite carbon blocks and learn more about (1) decarbonizing energy systems and industry, (2) thermal energy storage materials, and (3) storing heat in carbon blocks. Today, we shine a light on the transformational role carbon graphite blocks can play in powering our future sustainably.
Why do we care about energy storage?
Energy storage is the linchpin of decarbonizing our economy. We’ve figured out how to generate abundant renewable energy. However, energy demand does not always match supply. We may need energy when the sun isn’t shining or when the wind isn’t blowing, and storing intermittent renewable energy generation for use at times of higher demand will help us move away from fossil fuels. There are also cases of surplus energy wasted when, in very sunny places, excess solar cannot be added onto the grid, which needs to be balanced at all times.
The intermittency of renewable sources like the sun and wind presents a challenge. Enter energy storage. We're not only optimizing renewable energy generation but also paving the way for a zero-carbon grid. And as my friend Ben highlights in his recent article on batteries, there's a smorgasbord of technologies at our disposal. One form of energy storage technology I want to explore in this post is Thermal Energy Storage (TES).
How does thermal energy storage work?
Thermal energy storage generally involves accumulating energy in materials that allow it to be retained and released in a controlled manner. Abundant, inexpensive, and thermally stable materials such as carbon and molten salts provide an apealing alternative to rare earth mineral intensive batteries. Here’s a framework for different heat storage mediums
High temperature reservoir thermal energy storage systems use excess electricity to heat a storage medium to a high temperature, which can be used to generate electricity! Here we focus on solid state materials due to their high operating temperature and long storage duration. The key ideas in how solid state thermal storage works include specific heat capacity, temperature differentials, and the extraction of energy in the form of heat and power.
While we can’t cover all forms of TES in one article, I’m excited to explore one technology composed of a greatly abundant material on our planet: carbon. Yes, carbon. Abundant, cheap, and simple carbon. The same carbon that is in excess in our atmosphere, though instead of in its gaseous CO2 form, in its solid graphite (C) form. Compared to lithium-ion batteries, Antora's thermal energy storage is 90% cheaper for applications of 100+ hours of storage. TES can be added to our suite of storage solutions to improve efficiency, flexibility, and dispatchability of electricity.
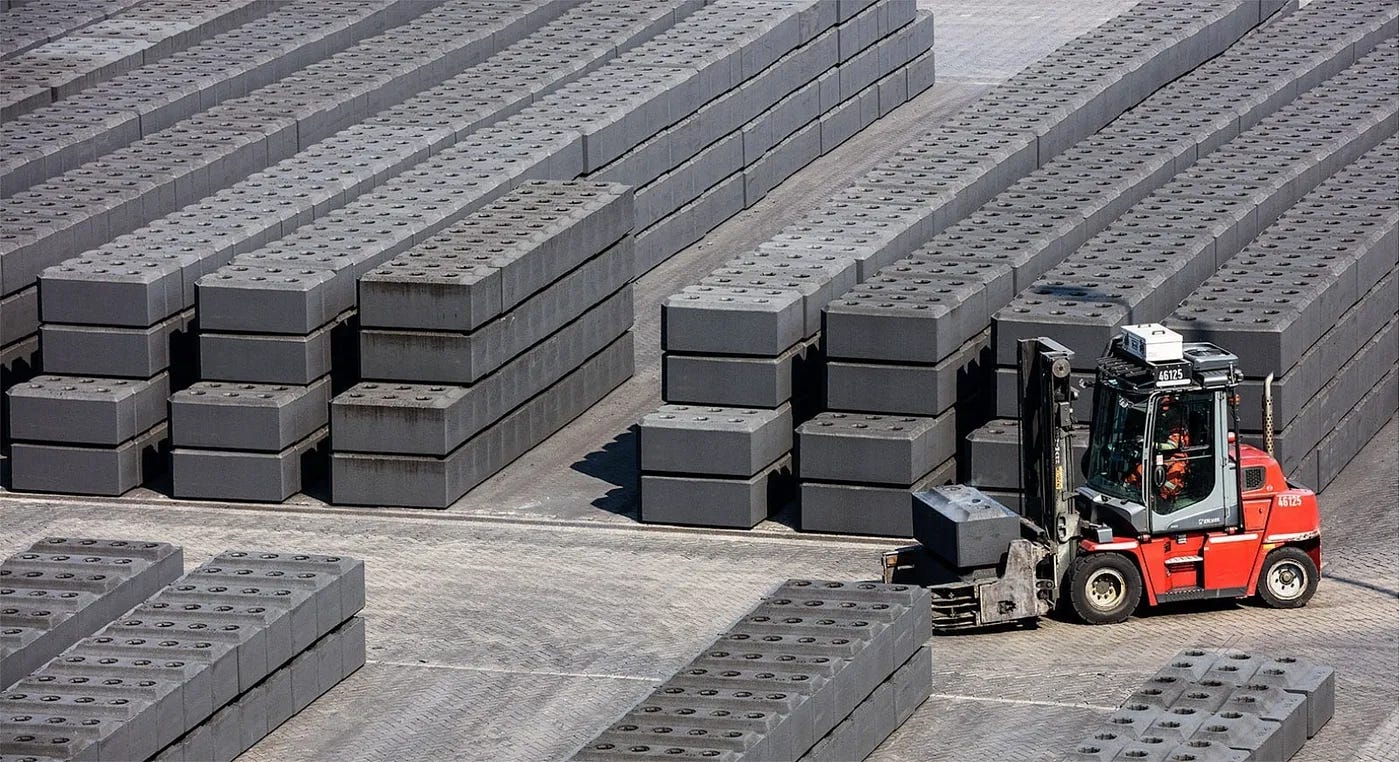
Graphite carbon is a solid-state storage medium. How much energy can be stored in carbon? The answer lies in energy density and specific heat capacity. Energy density for TES materials refers to how much energy can be stored per unit mass. For sensible heat energy storage,
energy density = mass ⋅ specific heat capacity ⋅ temperature differential
The thermal efficiency of sensible energy storage depends on the temperature differential between the hot and cold temperature heat reservoirs. In simpler terms, the difference between how hot and how cold you can get the material is the amount of energy that can be stored (and potentially extracted). The energy density is determined by the operating temperature difference. The maximum temperature carbon blocks can operate under is very high, meaning more energy can be stored.
The higher operating temperatures of solid-state media make it a strong candidate for providing clean heat for industrial processes (Fig. 6). The stored energy in carbon blocks can last for days to months with less than 2% heat loss for large scale systems, depending on the insulation.
Sensible heat storage is energy stored as temperature difference in solid or liquid media. The greater the difference between the hot and cold temperature reservoirs, the better.
Let’s get this bread–and heat and power
Whether from fossil fuels or renewables, generated heat must then be transferred to another process to be useful. Industrial process heat needs include preheating for boiler feedwater, hot water for various processes, hot air for drying, steam production, and direct heating.
How do we transfer heat stored in massive carbon bricks to what we actually want to heat? Heat transfer to the material can be direct or indirect. Direct transfer involves direct contact (ouch, hot stove) or heat via convection. Indirect heat transfer, on the other hand, utilizes a radiant heat transfer mechanism by routing hot gasses through radiant burner tubes or panels. By moving hot gasses through tubes, heat radiates off the tubes to the desired medium. A heat exchanger can be used to extract heat stored directly from the carbon blocks to the gases or liquids or other materials that need to be heated.
Antora Energy has developed the highest efficiency solid-state heat engine in history. Electricity can also be extracted from thermal energy storage mediums through Thermophotovoltaic (TPV) cells like the one Antora Energy is developing. Check out this article by Antora’s CEO, Turning sunshine and wind into 24/7 industrial heat and power — cheaper than fossil fuels, which does a better job of what I aim to describe here.
Why do we need thermal energy storage to decarbonize industry?
Industry refers to the process of making things like cement, steel and plastic, a major culprit of greenhouse gas emissions globally. A primary reason for this is that the high-temperature heat needed for industrial processes typically comes from natural gas among other fossil fuels.
Thermal energy storage (TES) can accelerate decarbonization as it can support long-duration energy storage and electrifying heat for industrial applications. The industrial processes reliant on fossil fuel combustion for high-temperature heat can be replaced by renewable electricity.
The industrial sector accounts for about a quarter of energy consumption and greenhouse gas (GHG) emissions in the U.S. Thermal processes make up 74% of the total energy usage in the industrial sector. Among these thermal processes, process heating accounts for 35%, combined heat and power for 26%, and conventional boilers for 13%. Electrifying process heat presents an exciting opportunity for decarbonization.
In simpler terms, industry — manufacturing plants for our steel and cars to cement for the foundation of our homes — requires materials (water, air, chemicals) to get really hot. How we get things hot today requires the burning of fossil fuels. Why is it so hard to get things hot in a sustainable manner? Solar, wind, and hydro all run turbines that generate electricity. The electricity then needs to be used to generate high temperatures. Thermal energy storage provides another efficient avenue in generating and storing clean electricity. Additional advantages of thermal energy storage include rapid implementation, ease of installment, and limited structural changes, enabling an affordable clean energy transition.
If we’re going to make it easier for our day to day lives to be more sustainable, we need systems level, infrastructural change. We want to build systems that allow each of us to turn the lights on with the peace of mind that the sources of electricity are non-polluting nor contributing to climate change. We want to build a system where the sustainable option is the default option. Decarbonizing the energy sector which powers our day to day lives enables this.
Okay, I’ll stop here for now. I hope you’ve enjoyed this article of Daylight Climate! With this spotlight on thermal energy storage through carbon blocks, I hope you feel more optimistic about how we might decarbonize “hard-to-abate” sectors like industry. Bask in the sun’s warmth, and enjoy the last few hours of the long weekend.
Sending good energy,
Lisa
References
IRENA (2020), Innovation Outlook: Thermal Energy Storage, International Renewable Energy Agency, Abu Dhabi. https://www.irena.org/publications/2020/Nov/Innovation-outlook-Thermal-energy-storage
Fifty Years News Contributors. (2022, February 16). Antora Energy. Fifty Years News. https://fiftyyears.substack.com/p/antora-energy
Hasanbeigi, Ali, et al. (2021). Electrifying U.S. Industry: A Technology and Process-Based Approach to Decarbonization.
Ponec, A. (2022, February 19). Turning sunshine and wind into 24/7 industrial heat and power — cheaper than fossil fuels. Medium. https://medium.com/antora-energy/turning-sunshine-and-wind-into-24-7-industrial-heat-and-power-cheaper-than-fossil-fuels-69355cdcde04
Tamme, R., Laing, D., Steinmann, W.-D., & Bauer, T. (n.d.). Thermal Energy Storage. In Encyclopedia of Sustainability Science and Technology (pp. 10551–10577). Springer New York. https://doi.org/10.1007/978-1-4419-0851-3_684
Tetteh, S., Yazdani, M. R., & Santasalo-Aarnio, A. (2021). Cost-effective Electro-Thermal Energy Storage to balance small scale renewable energy systems. Journal of Energy Storage, 41, 102829. https://doi.org/10.1016/j.est.2021.102829
(2023, May 2). Why it might be simpler than thought to decarbonise industry. World Economic Forum. https://www.weforum.org/agenda/2023/05/decarbonising-industry-renewable-electricity-thermal-energy-storage/?